<b>Fennel</b>, K., S. R. Alin, L. Barbero, W. Evans, T. Bourgeois, S. R. Cooley, J. Dunne, R. A. Feely, J. M. Hernandez-Ayon, C. Hu, X. Hu, S. E. Lohrenz, F. Muller-Karger, R. G. Najjar, L. Robbins, J. Russell, E. H. Shadwick, S. Siedlecki, N. Steiner, D. Turk, P. Vlahos, and Z. A. Wang, 2018: Chapter 16: Coastal ocean and continental shelves. In Second State of the Carbon Cycle Report (SOCCR2): A Sustained Assessment Report [Cavallaro, N., G. Shrestha, R. Birdsey, M. A. Mayes, R. G. Najjar, S. C. Reed, P. Romero-Lankao, and Z. Zhu (eds.)]. U.S. Global Change Research Program, Washington, DC, USA, pp. 649-688, https://doi.org/10.7930/ SOCCR2.2018.Ch16.
Coastal Ocean and Continental Shelves
Carbon is present in various inorganic and organic forms in coastal waters (see Figure 16.2). Dissolved inorganic species include aqueous CO2 (a combination of dissolved CO2 and carbonic acid), bicarbonate and carbonate ions, and methane (CH4); the first three carbon species are collectively referred to as dissolved inorganic carbon or DIC. The major particulate inorganic species is calcium carbonate (CaCO3), also referred to as particulate inorganic carbon (PIC). Carbon is also present in various dissolved and particulate organic forms (DOC and POC). In shelf waters, the reduced carbon pool or total organic carbon pool (TOC) represents roughly 2% to 5% of the total carbon stock (Liu et al., 2010), and DOC constitutes more than 90% to 95% of this TOC (Vlahos et al., 2002).
Figure 16.2: Major Coastal Carbon Pools and Fluxes
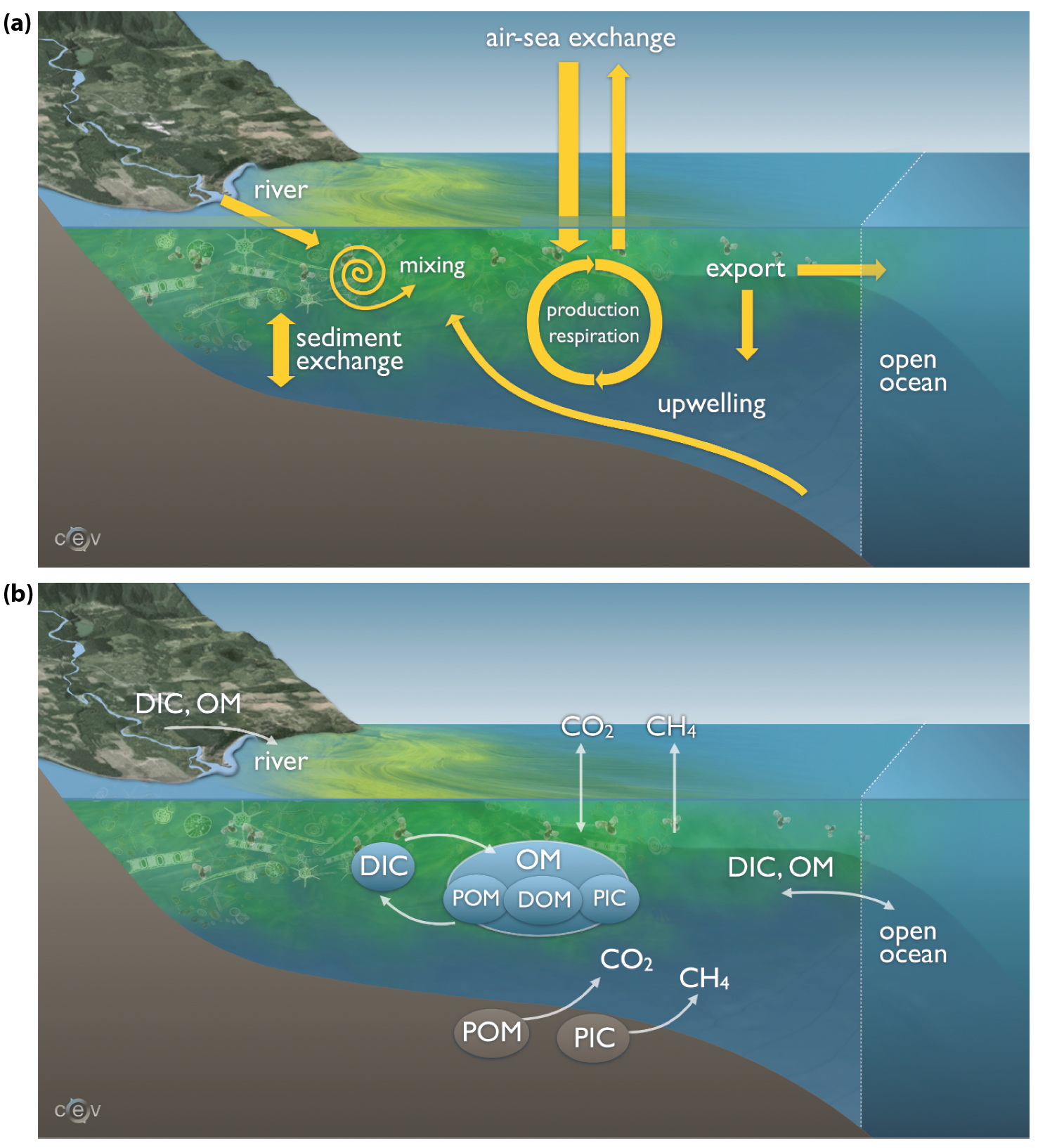
Carbon is constantly transferred among these different pools and exchanged across the interfaces that demarcate coastal waters: the land-ocean interface, the air-sea interface, and the interface between coastal and open-ocean waters (see Figure 16.2). The internal carbon transformations within coastal regions include photosynthetic primary production, respiration, transfers between lower and higher trophic levels of the food web, exchanges between sediment and overlying water, biogeochemical processes in the sediment, and the formation and dissolution of CaCO3. Major internal transformations are the conversion of DIC into organic carbon (POC and DOC), through primary production, and respiration throughout the water column, returning most of the organic carbon into inorganic forms (primarily DIC). Some POC settles out of the water column and becomes incorporated into the sediments where most of this material is respired through a range of different redox processes that produce DIC and, under certain circumstances, CH4 (i.e., in the relative absence of electron acceptors other than CO2). Both DIC and CH4 are released back into the overlying water. POC that is not respired (referred to as refractory POC) can be buried in sediments and stored for a very long time. Some organisms form internal or external body structures of CaCO3, which either dissolve or become incorporated into the sediments and are buried. This discussion will refer to long-term storage of buried POC and PIC in coastal sediments as permanent burial.
A major carbon exchange process along the ocean margin is the flux of CO2 across the air-sea interface. The annual cycle of this flux is driven by 1) seawater warming and cooling, which affects CO2 solubility; 2) the under- or oversaturation of CO2 resulting from primary production, respiration, and CaCO3 precipitation and dissolution; 3) the transport of DIC to and from the ocean surface (e.g., upwelling and convection); and 4) factors that influence the resistance to gas exchange across the air-sea interface (e.g., winds, sea ice extent, and surface films). The annual cycles of primary production, respiration, and air-sea CO2 flux tend to be of larger magnitude and more variable in coastal waters than in the open ocean (Bauer et al., 2013; Liu et al., 2010; Muller-Karger et al., 2005; Thunell et al., 2007; Xue et al., 2016) and more pronounced in high latitudes. Other important exchange fluxes are organic and inorganic carbon inputs from land via rivers and estuaries (see Ch. 15: Tidal Wetlands and Estuaries), from tidal wetlands, and exchanges between the coastal and open oceans across the continental shelf break or the operationally defined open-ocean boundary of the coastal ocean. Net removal of carbon from direct interaction with the atmospheric reservoir can occur by export to the deep ocean or by permanent burial in coastal sediments.
Although continental shelves make up only 7% to 10% of the global ocean surface area, they are estimated to contribute up to 30% of primary production, 30% to 50% of inorganic carbon burial, and 80% of organic carbon burial (Dunne et al., 2007; Gattuso et al., 1998). As such, continental shelves have been argued to contribute disproportionately to the oceanic uptake of CO2 (Cai 2011; Liu et al., 2010; Muller-Karger et al., 2005).
Carbon export, referring to the flux of organic and inorganic carbon from coastal waters to the deep ocean, can occur through the so-called “Continental Shelf Pump”—a term coined by Tsunogai et al. (1999) after they observed a large uptake of atmospheric CO2 in the East China Sea. There are two distinct mechanisms underlying the Continental Shelf Pump (Fennel 2010). The first is physical in nature and thought to operate in mid- and high-latitude systems. In winter, shelf water is cooled more strongly than surface water in the adjacent open ocean because the former is not subject to deep convection. The colder shelf water is denser and experiences a larger influx of atmospheric CO2; both density and the solubility of CO2 increase with decreasing temperature. If this dense and carbon-rich water is transported off the shelf, it will sink due to its higher density, and the associated carbon will be exported to the deep ocean. The second mechanism relies on biological processes that concentrate carbon below the seasonal pycnocline (i.e., photosynthetic production of organic carbon and subsequent sinking). If the carbon-rich water below the seasonal pycnocline is moved off the shelf horizontally, carbon potentially could be exported if this water is transported or mixed below the seasonal thermocline. The depth to which the shelf-derived carbon can be exported will be different for POC, which will sink, and DOC and DIC, which primarily would be advected laterally. Both mechanisms for carbon export critically depend on physical transport of carbon-rich water off the shelf.
Carbon export flux from coastal waters to the deep ocean cannot be quantified easily or accurately through direct observation. Thus, the only available estimates of such export are indirect, using mass balances of POC and dissolved oxygen (Hales et al., 2006), mass balances of DOC (Barrón and Duarte 2015; Vlahos et al., 2002), mass balances of TOC and DIC (Najjar et al., 2018), and model estimates (Izett and Fennel 2018a, 2018b; Bourgeois et al., 2016; Fennel and Wilkin 2009; Fiechter et al., 2014; Mannino et al., 2016; Turi et al., 2014; Xue et al., 2013). If the total carbon inventory in a coastal system can be considered constant over a sufficiently long timescale (i.e., on the order of years), inferring carbon export is possible from using the sum of all other exchange fluxes across the system’s interfaces over that same period. Export to the open ocean must balance the influx of carbon from land and wetlands, its net exchange across the air-sea interface, lateral exchange caused by advection, and any removal through permanent sediment burial. The accuracy of the inferred export flux directly depends on the accuracy of the other flux estimates and of the assumption of a constant carbon inventory. Quantifying internal transformation processes (e.g., respiration and primary and secondary production) does not directly enter this budgeting approach but can elucidate the processes that drive fluxes across interfaces.
Current estimates of carbon fluxes across coastal interfaces come with significant uncertainties (Regnier et al., 2013; Birdsey et al., 2009). These uncertainties are caused by a combination of 1) small-scale temporal and spatial variability, which is undersampled by currently available means of direct observation, and 2) regional heterogeneity, which makes scaling up observations from one region to larger areas difficult. Contributing to variability in regional carbon budgets and export are geographical differences arising from variations in shelf width, the presence or absence of large rivers, seasonal ice cover, and latitude through its modulation of annual temperature and productivity cycles and of hydrography due to the rotation of the Earth (Sharples et al., 2017). Section 16.3 describes the regional characteristics of North American coastal waters and how these characteristics influence carbon dynamics. Available estimates of carbon fluxes are compiled in an attempt to estimate export.
The motivation for quantifying permanent burial of carbon and export of carbon from coastal waters to the deep ocean is that both processes remove CO2 from the atmospheric reservoir. A more relevant but harder to obtain quantity in this context is the burial or export of anthropogenic carbon. The anthropogenic component of a given carbon flux is defined as the difference between its preindustrial and present-day fluxes. Thus, present-day carbon fluxes represent a superposition of the anthropogenic flux component and the natural background flux. Only total fluxes—the sum of anthropogenic and background fluxes—can be observed directly. Distinction between anthropogenic fluxes and the natural background is difficult to assess for coastal ocean fluxes and has to rely on process-based arguments and models (Regnier et al., 2013). Observation-based estimates of the global open ocean’s anthropogenic uptake have been made by Sabine et al. (2004), Sabine and Tanhua (2010), and Carter et al. (2017). Bourgeois et al. (2016) were the first to estimate coastal anthropogenic carbon uptake in their global model. Their estimates are presented in some detail in Section 16.3.5.
See Full Chapter & References